Phase-contrast and scattering-based dark field X-ray imaging can potentially revolutionize the radiological approach to medical imaging because they are intrinsically capable of detecting subtle differences in the electron density of a material and of measuring the effective integrated local small-angle scattering power generated by the microscopic density fluctuations in the specimen.
Grating Interferometry
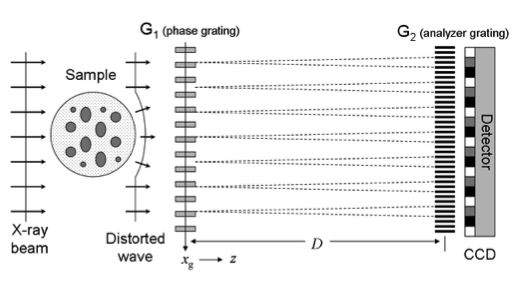
The principle of imaging with a grating interferometer is shown in Figure 1. A conventional interferometer consists of a phase grating, G1, and an absorption grating, G2. The first grating (G1) acts as a beam splitter and essentially divides the incoming beam into the two first diffraction orders. Since the wavelength of the illuminating hard X-rays (~10-10 m) is much smaller than the grating pitch (~10-6 m), the angle between the two diffracted beams is so small that they overlap almost completely. Downstream of the beam-splitter grating, the diffracted beams interfere and form linear periodic fringe patterns in planes perpendicular to the optical axis. Perturbations of the incident wave front, such as those induced by refraction by a phase object in the beam, lead to local displacement of the fringes. The basic idea of the grating interferometry (GI) method is to detect the positions of the fringes and from these determine the shape of the wave front. However, since the periodicity of the phase grating (and thus the spacing of the interference fringes) does not exceed a few microns, an area detector placed in the detection plane will generally not have sufficient resolution to resolve the fringes, i.e., the exact position of their maxima. Therefore, a grating, G2, with absorbing lines and the same periodicity and orientation as the fringes, is placed in the detection plane immediately before the detector. This analyser grating acts as a transmission mask for the detector and transforms local fringe positions into signal-intensity variations. The detected signal profile thus contains quantitative information about the phase gradient of the object. Small angle scattering provides complementary and otherwise inaccessible structural information about the sample at the micrometer and sub-micrometer length scale. This contrast mechanism is also known as dark-field (DF) imaging, in analogy to visible-light Fourier optics. The amplitude of the first Fourier component of the intensity signal is decreased when X-rays are scattered or reflected at internal interfaces on their way through the specimen. The small-angle scattering signal as recorded in the X-ray dark-field image is particularly sensitive to density variations in the object on the length scale of a few tenths of nanometres to several micrometres. It naturally complements the length scales that can be imaged directly by radiographic methods into the sub micrometre range. As a consequence, a grating interferometer can be efficiently used to simultaneously produce dark-field, phase contrast, and conventional absorption images with hard X-rays.
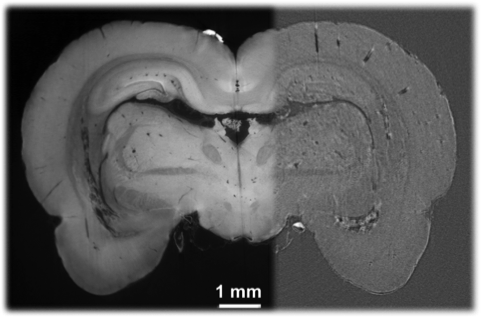
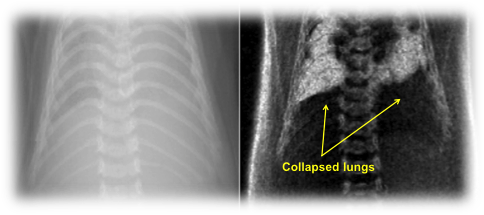
GI-based breast imaging
Grating-based interferometry (GI) has emerged as a very suited approach to introduce phase- and dark-field X-ray imaging into clinic. Seminal works on early breast cancer detection (on mastectomies) and lung imaging (on mice and pigs) have paved the road for GI to become the disruptive X-ray diagnostic tool of the future. Figure 4 shows a schematic setup of a GI-breast imaging: a first grating (G0) is used to generate coherent X-ray to illuminate the patient, a second grating (G1) introduces a reference interference pattern and the last grating (G2) enables to read out changes in the interference pattern induced by the sample. This yields to three complementary images, as shown in Figure 5.
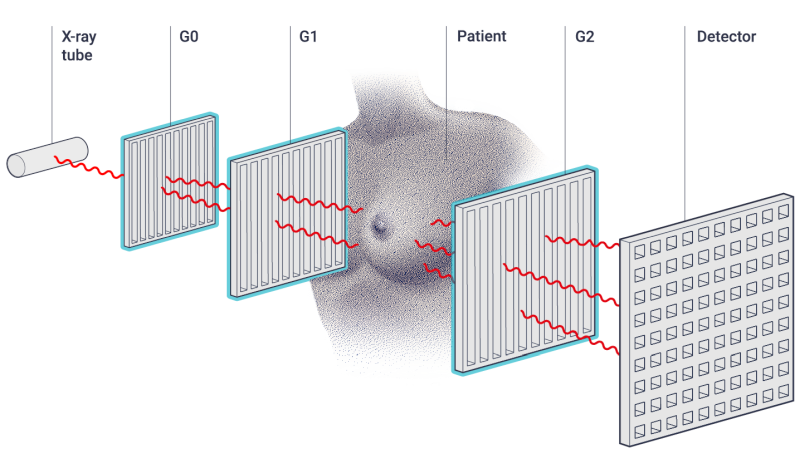
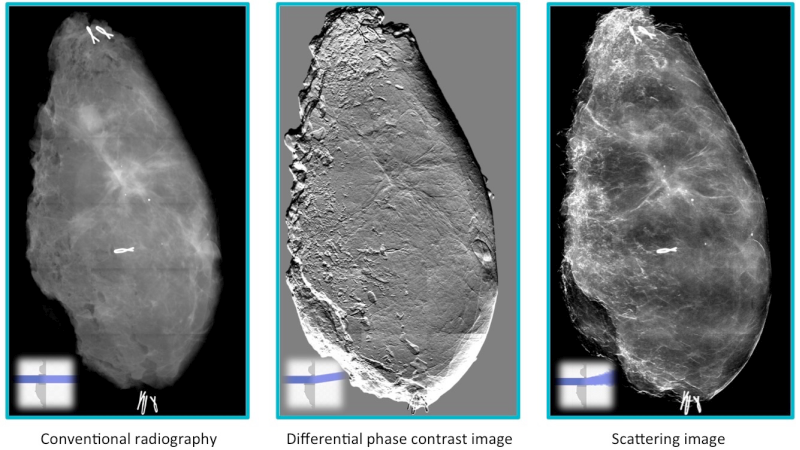